Chapter 8 Geological Resources
Learning Objectives
After reading this chapter, completing the exercises within it, and answering the questions at the end, you should be able to:
- Describe the importance of geological resources to our way of life,
- Explain some of the processes involved in the formation of metal deposits,
- Explain how mines are made and why mining can lead to generation of acid rock drainage and contamination of the environment by metals,
- Summarize some of the important industrial materials extracted and describe what they are used for,
- Describe the processes that lead to the formation of coal deposits,
- Explain the processes that lead to the formation of oil and gas, the distinction between source rocks and reservoir rocks and the importance of traps,
- Understand the climate implications of producing and using fossil fuels, and
- Understand the implications of mining and mineral processing for the climate and for Earth systems processes.
It has been said that “if you can’t grow it, you have to mine it”, meaning that everything that we use and can’t grow, has to be extracted from Earth in one way or another. This includes water, of course— our most important resource—but it also includes all the other materials that we use to construct things like roads, dams and bridges, manufacture things like teacups, toasters, and telephones; and energy, although our days of removing fossil fuels stored in the crust must end very soon.
Virtually everything that we use every day is made from resources from the Earth. For example, let’s look at a tablet computer (Figure 8.0.1). Most of the case is made of a plastic known as ABS which is made from either gas or petroleum. Some tablets have a case made from aluminum. The glass of a touch screen is made mostly from quartz combined with smaller amounts of sodium oxide (Na2O), sodium carbonate (Na2CO3), and calcium oxide (CaO). To make it work as a touch screen the upper surface is coated with indium tin oxide. When you touch the screen you’re actually pushing a thin layer of polycarbonate plastic (made from petroleum) against the coated glass—completing an electrical circuit. The computer is able to figure out exactly where you touched the screen. Computer processors are made from silica wafers (more quartz) and also include a significant amount of copper and gold. Gold is used because it is a better conductor than copper and doesn’t tarnish the way silver does. Most modern electronic devices and computers have Lithium-ion polymer batteries, which include aluminum, copper, nickel, cobalt, iron, manganese, lithium and graphite. The processor and other electronic components are secured to a circuit board which is a thin layer of fibreglass sandwiched between copper sheets that are coated with small amounts of tin and lead. Various parts are put together with steel screws that are made mostly of iron and molybdenum.
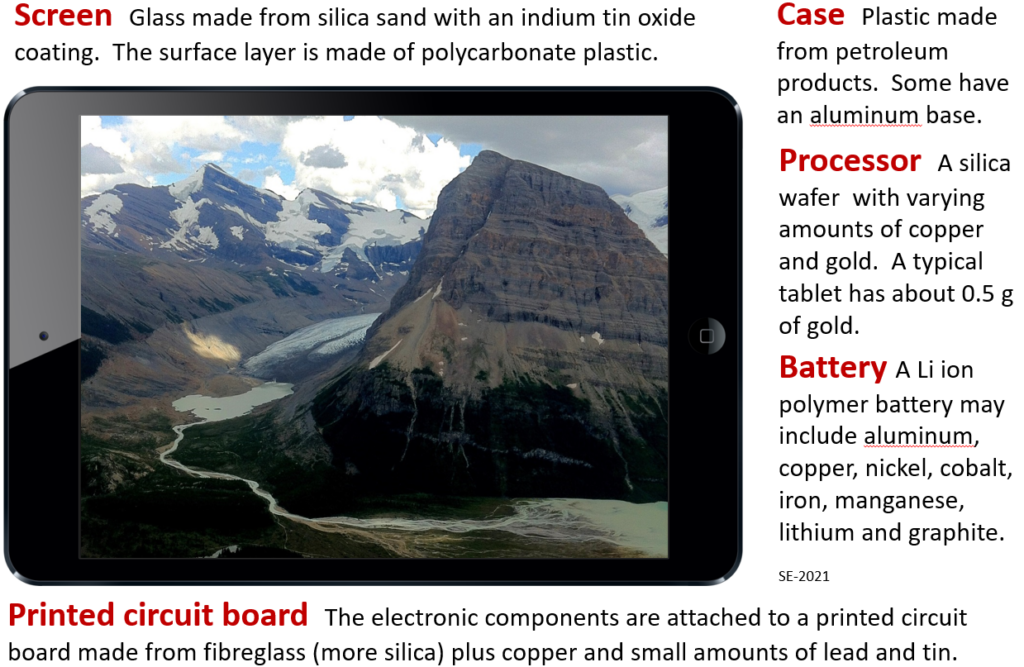
That’s not everything that goes into a tablet computer, but to make just those components we need a pure-silica sand deposit, a salt mine for sodium, a rock quarry for calcium, an oil well, a gas well, an aluminum mine, an iron mine, a manganese mine, a copper-molybdenum-gold mine, a cobalt-nickel mine, a lithium mine, and a source of energy to transport all of the materials, process and put them together, and finally to transport the computer to your house or the store where you bought it.
Exercise 8.1 Where Does it Come From?
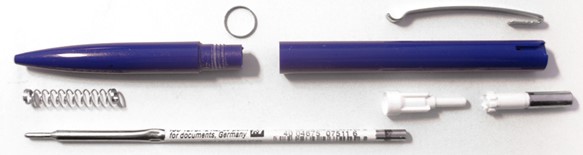
Look around you and find at least five objects (other than a computer or a phone) that have been made from materials that had to be mined, quarried or extracted from an oil or gas well. Try to identify the materials involved, and think about where they might have come from. Figure 8.0.2, showing the parts of a retractable ball-point pen, is just an example.
Batteries are becoming increasingly common in our lives, not just to power small things like phones and computers, but increasingly cars, ships, and even aircraft, and huge battery banks are also becoming important components of our electricity grids. Although battery technology is evolving quickly, lithium-ion batteries (Figure 8.0.3) are still the ones to beat for power to weight performance, safety and repeated charging ability, and so there is an increasing demand for lithium and for the other metals that are used in lithium-ion batteries, particularly copper, nickel and cobalt). In the latter part of the 20th century the global demand for lithium was in the range of 1,000 to 3,000 tonnes per year. By 2010 that had reached 30,000 t/y, and by 2020 closer to 100,000 t/y. Growth in demand is has been doubling every 4 to 5 years for the past two decades, and that trend is expected to continue for at least the next decade.[1][2]
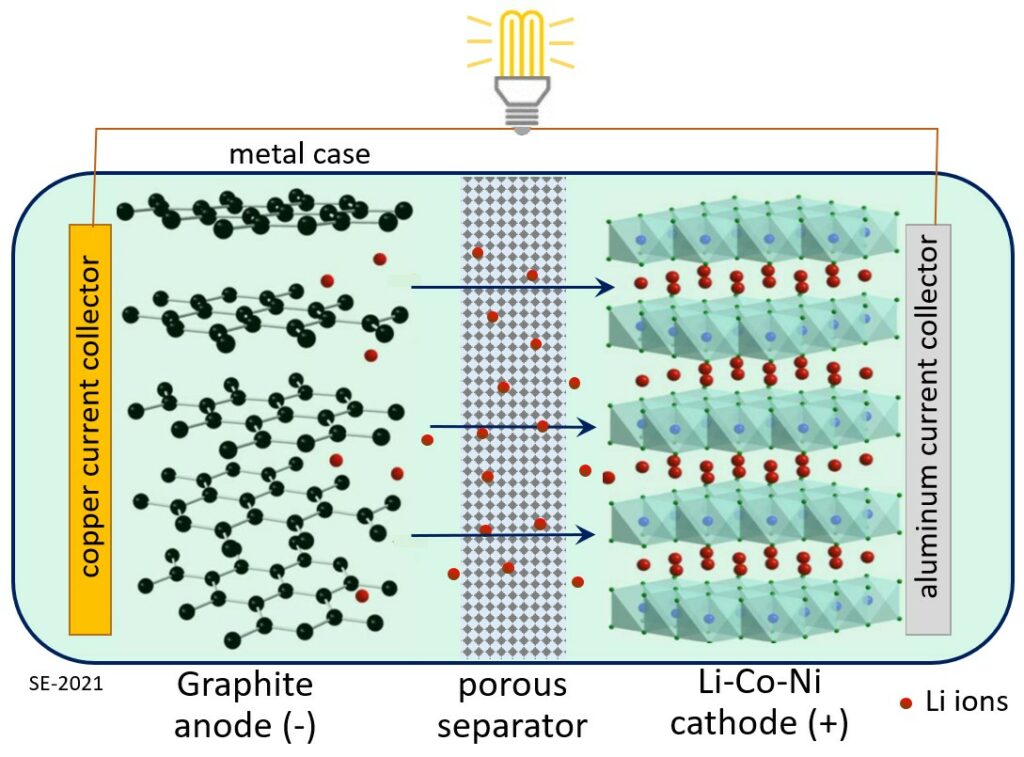
Although most of the world’s current lithium production comes from hard-rock mines in Australia, the greatest known lithium reserves are in salt lakes in Bolivia, Argentina and Chile (Figure 8.0.4).
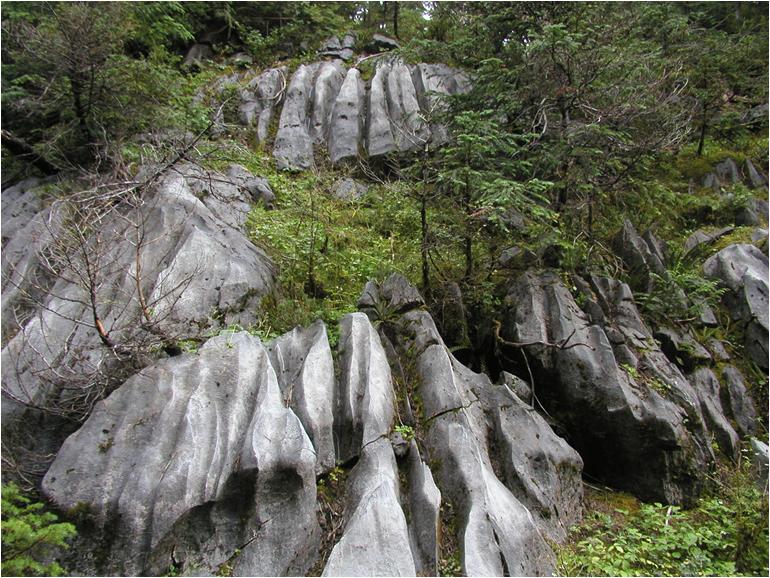
Brines in solar lakes typically have lithium ion concentrations in the order of 0.06 to 0.16%, but they also contain a number of other ions, especially Ca, Mg, and sulphate that need to be removed before the lithium can be recovered. This removal is accomplished by adding phosphate and carbonate that will preferentially form salts with Ca and Mg, leaving the lithium in solution. The brine is then concentrated by evaporation in several stages (hence the different-coloured lagoons visible in Figure 8.0.3), and finally the lithium is separated by addition of carbonate and formation of LiCO3.[3]
Media Attributions
- Figure 8.0.1 Steven Earle, CC BY-SA 3.0; based on IPad Air by Zach Vega, CC BY-SA 3.0 via Wikimedia Commons, https://upload.wikimedia.org/wikipedia/commons/8/8d/IPad_Air.png
- Figure 8.0.2 Ballpoint Pen Parts by Pavel Krok, 2005, CC BY SA 2.5, via Wikimedia Commons, https://commons.wikimedia.org/wiki/File:Ballpoint-pen-parts.jpg
- Figure 8.0.3 Steven Earle, CC BY 4.0
- Figure 8.0.4 Lithium Mine at Uyuni Salt Flat by Coordenação-Geral de Observação da Terra/INPE, 2018, CC BY-SA 2.0, via Wikimedia Commons, https://commons.wikimedia.org/wiki/File:Lithium_mine_at_Bolivia%C2%B4s_Uyuni_Salt_Flat,_on_a_CBERS4_MUX_yesterday’s_image.jpg
- Lu, S. & Frith, J. (2019). Will the real lithium demand please stand up? Challenging the 1Mt-by-2025 Orthodoxy. Bloomberg NEF. https://about.bnef.com/blog/will-the-real-lithium-demand-please-stand-up-challenging-the-1mt-by-2025-orthodoxy/ ↵
- See Office of Energy Efficiency & Renewable Energy. (2017). How does a lithium-ion battery work? https://www.energy.gov/eere/articles/how-does-lithium-ion-battery-work for an overview of the function of a Li-ion battery. ↵
- Woon An, J., et al. (2012). Recovery of lithium from Uyani salar brine, Hydrometallurgy, 117-118, 64-70. https://doi.org/10.1016/j.hydromet.2012.02.008 ↵