6.5 Forecasting Earthquakes and Minimizing Damage and Casualties
Steve Earle
It has long been a dream of seismologists, geologists and public safety officials to be able to accurately predict earthquakes on time scales (weeks, days or hours) that would be useful for minimizing danger to the public and damage to infrastructure. Many different avenues have been explored, such as warning foreshocks, changes in magnetic fields, seismic tremor, changing groundwater levels, strange animal behaviour, observed earthquake periodicity, stress transfer considerations and a range of others. So far, none of the research into earthquake prediction has provided a reliable method. Although there are reports of successful earthquake predictions, they are rare, and many are surrounded by doubtful circumstances.
The problem with earthquake predictions, as with any other type of prediction, is that they have to be accurate most of the time, not just some of the time. We have come to rely on weather predictions because they are generally (and increasingly) accurate. But if we try to predict earthquakes and are only accurate 10% of the time (and even that isn’t likely with the current state of knowledge), the public will lose faith in the process very quickly, and then all of the predictions will be ignored.
There was a great hope for earthquake predictions late in the 1980s, when attention was focused on part of the San Andreas fault at Parkfield, about 200 km south of San Francisco. Between 1881 and 1966 there were 5 earthquakes at Parkfield, most spaced at approximately 20-year intervals, all confined to the same 20 km-long segment of the fault and all very close to M6 (Figure 6.5.1). Both the 1934 and 1966 earthquakes were preceded by small foreshocks exactly 17 minutes before the main quake.
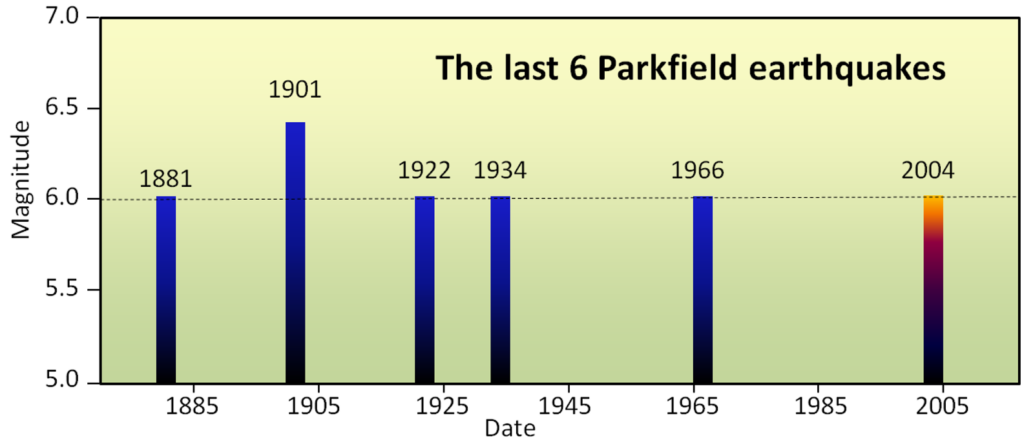
The U.S. Geological Survey recognized this as an excellent opportunity to understand earthquakes and earthquake prediction, so they armed the Parkfield area with a huge array of geophysical instruments and waited for the next quake, which was expected to happen around 1987. Nothing happened! The “1987 Parkfield earthquake” finally struck in September 2004, about 17 years late. Fortunately, all of the equipment was still there, but it was to no avail from the perspective of earthquake prediction. There were no significant precursors to the 2004 Parkfield earthquake in any of the parameters measured, including: seismicity, harmonic tremor, strain (rock deformation), magnetic field, the conductivity of the rock or creep, and there was no foreshock. In other words, even though every available technique was used to monitor it, the 2004 Parkfield earthquake came as a complete surprise, with no warning whatsoever.
The hope for earthquake prediction is not dead, but it was hit hard by the Parkfield experiment. The current focus in earthquake-prone regions is to provide forecasts of earthquake probabilities within a certain time period—typically a number of decades—and also to ensure that the population is educated about earthquake risks and that buildings and other infrastructure are as safe as can be. An example of this approach for the San Francisco Bay region of California is shown on Figure 6.5.2. Based on a wide range of information, including past earthquake history, accumulated stress from plate movement, and known stress transfer, seismologists and geologists have predicted the likelihood of a M6.7 or greater earthquake on each of 8 major faults that cut through the region. The greatest probabilities are on the San Andreas, Rogers Creek and Hayward faults. As shown on the diagram, there is a 72% chance that a major and damaging earthquake will take place somewhere in the region prior to 2043.
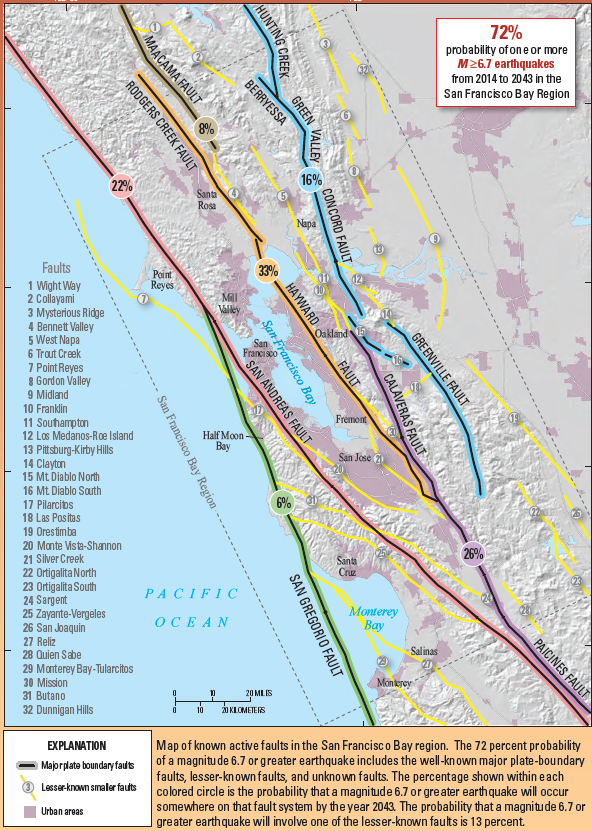
It is feasible to provide useful warnings of earthquakes that have already happened to urban areas that are some distance from the epicentre. As described in Section 6.4, the earthquake in Mexico in 1985 took place near to the coast but had devastating effects in Mexico City, several hundred kilometres away. In 1993 the Mexican Government established an early warning system for the capital. It consists of a network of seismometers along the coast that will relay an electronic warning to emergency officials in Mexico City who then activate alert systems in hospitals and schools, and public alarms on 12,000 pole-mounted speakers throughout the city. Because seismic waves can take well over a minute to reach Mexico City from the coast, and because the warning messages are almost instant, residents of the capital typically have up to two minute’s warning that an earthquake has happened and shaking can be expected. According to Cochran et al (2018) the system has wide public acceptance, even though many of the earthquakes that have been warned of were too small to cause any damage in Mexico City.
Japan established a nation-wide earthquake warning system in 2008 , and the US Geological Survey started work on the ShakeAlert system for Washington, Oregon and California in 2016, although it will be some years before that system is fully functional. Both of these systems are based on seismometers on land.
A unique earthquake early warning system, that uses seismometers on the sea floor, is being developed in British Columbia. The initiative is based on Ocean Networks Canada existing fibre-optics communication and instrumentation array on the sea floor off the west coast of Vancouver Island (Figure 6.5.3). Eight seismometers situated up to 200 km offshore, networked with roughly 100 seismometers on land, can detect a large earthquake up to 90 seconds before the shaking could reach major centres such as Vancouver and Victoria .
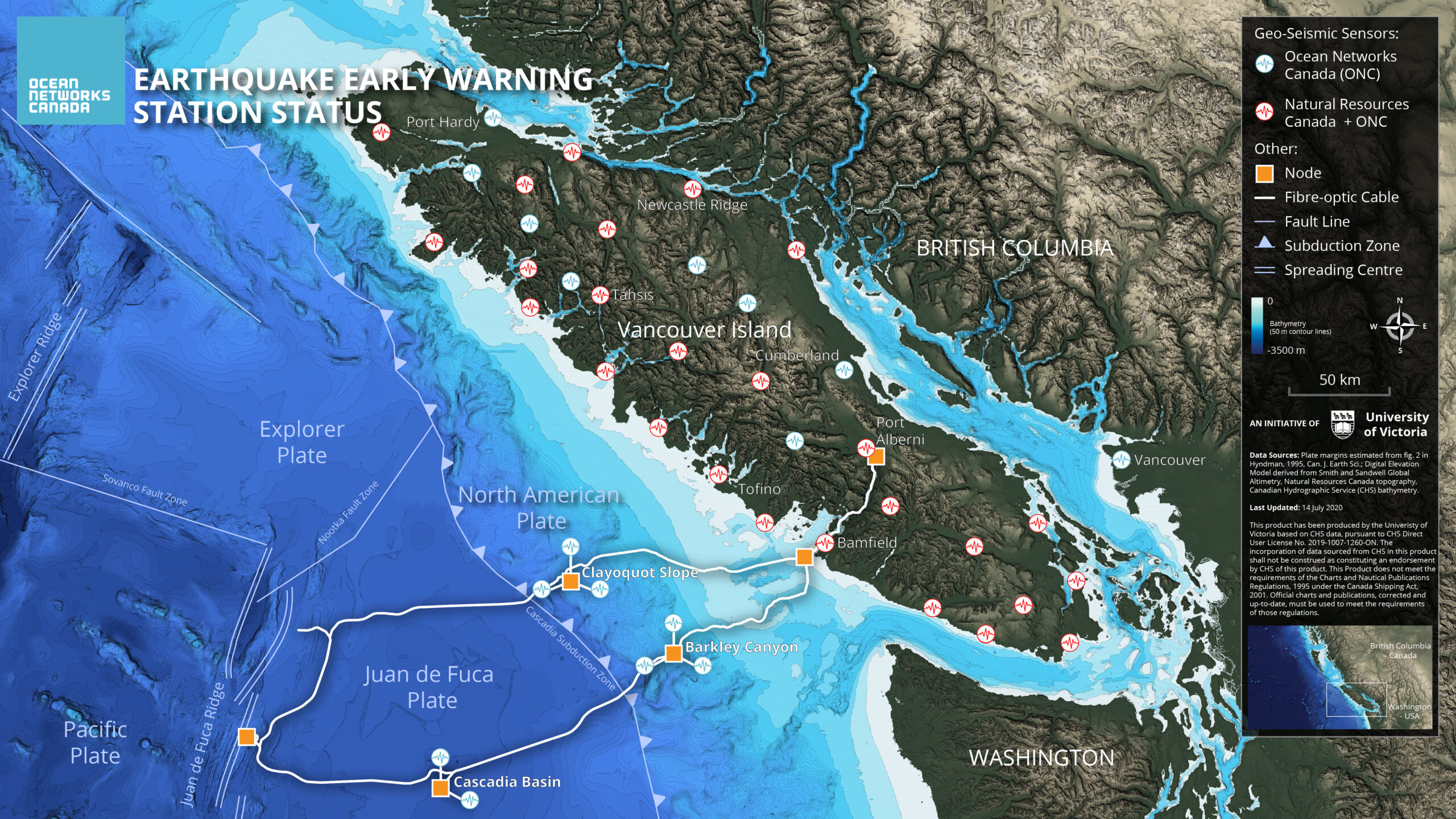
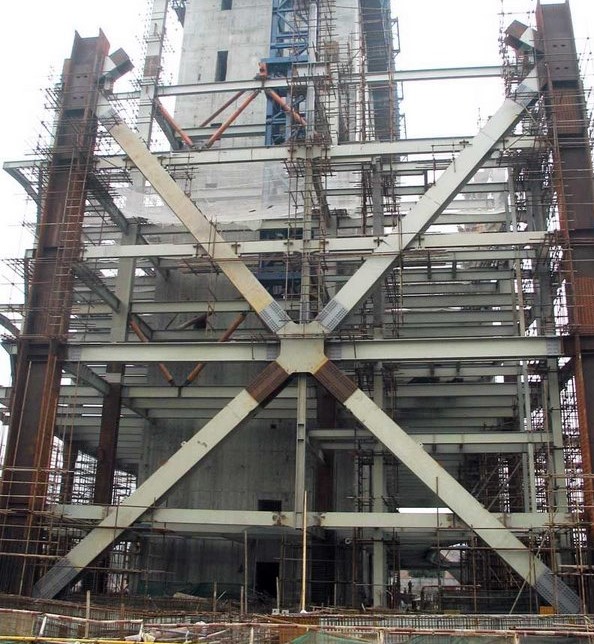
A critical component of reducing the damage and casualties from earthquakes is to ensure that buildings and other infrastructure (bridges, dams, roads etc.) are built to withstand strong shaking. This starts from ensuring that the foundation is constructed on strong material, and continues right up to ensuring that furniture and movable items (bookcases, water heaters etc.) are secure. Buildings themselves can be constructed to resist and withstand shaking by including diagonal bracing (Figure 6.5.4) and flexible foundations. Wooden buildings tend to perform well during shaking because of the flexibility of the wood. Bridges can also be designed to resist shaking. An interesting example of a bridge designed to withstand earthquakes is illustrated on Figure 6.5.5.
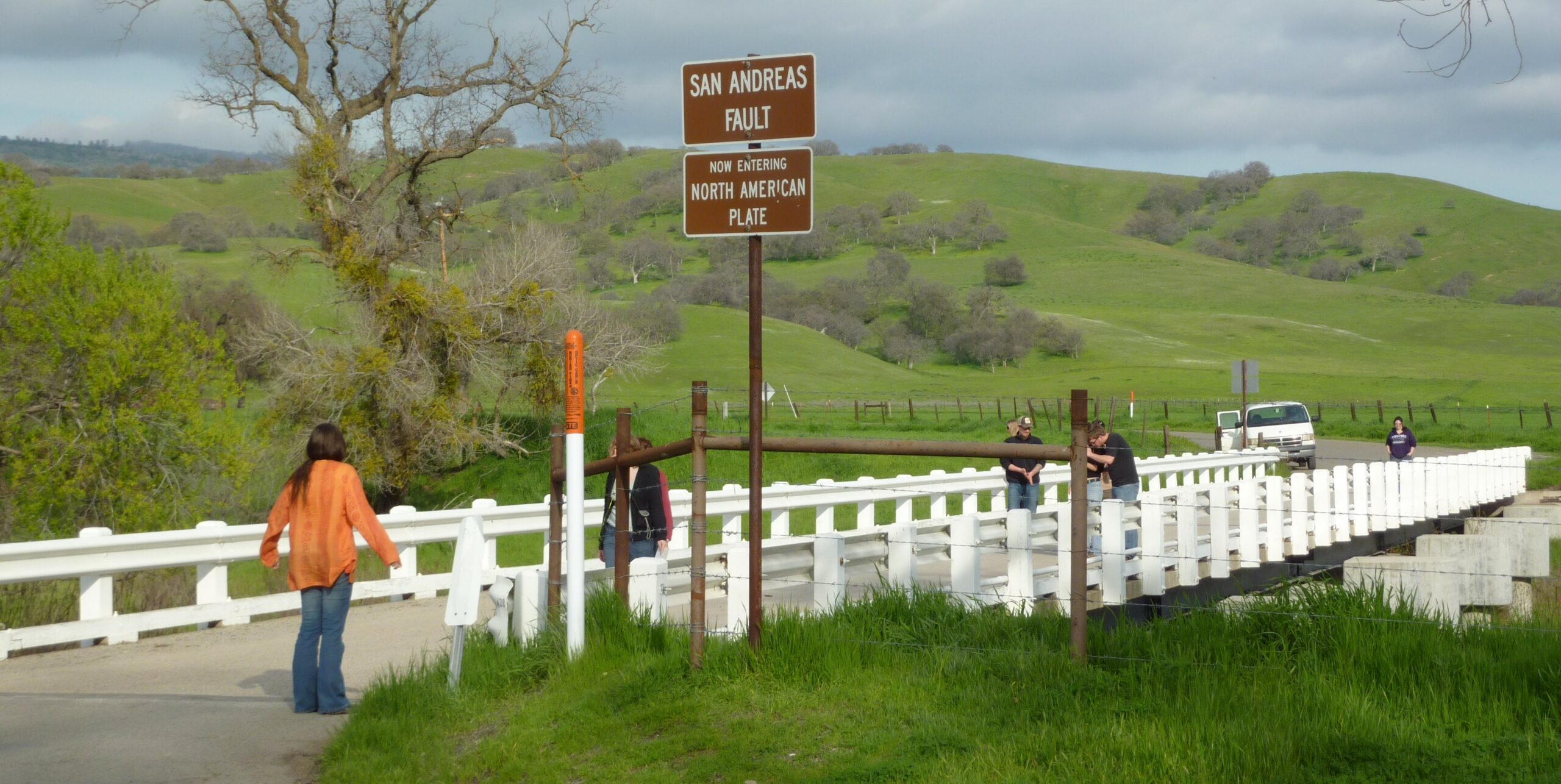
As we’ve discussed already, it’s not sufficient to have strong building codes, they have to be enforced. Building code compliance is quite robust in most developed countries, but is not adequate in many developing countries.
It’s also not enough just to focus on new buildings, we have to make sure that existing buildings— especially schools and hospitals—and also other structures such as bridges and dams, are as safe as they can be. Efforts to upgrade the safety of schools in British Columbia are described in Box 6.2.
Box 6.2 Making the Seismic Up-grade in BC’s schools
British Columbia is in the middle of a multi-billion dollar program to make schools safer for students. The program is focused on older schools, because, according to the government, those built since 1992 already comply with modern seismic codes. Some schools would require too much work to make upgrading economically feasible and they are replaced. Where upgrading is feasible, the school is assessed carefully before any upgrade work is initiated.
An example is Sangster Elementary in Colwood on southern Vancouver Island (Figure 6.5.6). The school was originally built in 1957, with a major addition in 1973. Ironically, the newer part of the school, built of concrete blocks, required strengthening with the addition of a steel framework, while the 1957 part, which is a wood-frame building, did not require seismic upgrading. The work was completed in 2014.
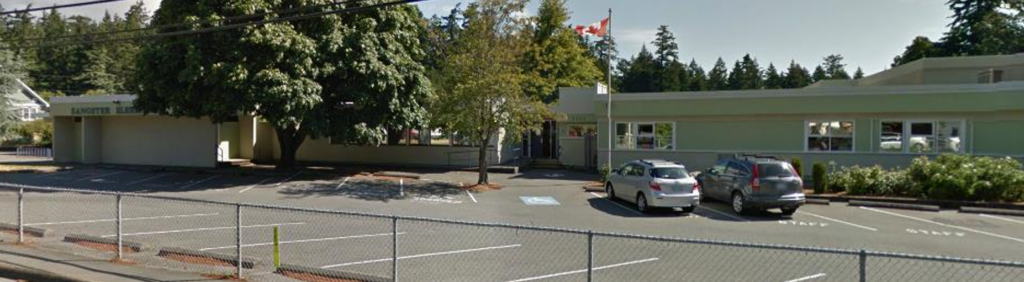
As of May 2021 upgrades had been completed at 186 B.C. schools, 30 were underway, and an additional 13 were ready to proceed with funding identified. Another 267 schools were listed as needing upgrades[1].
The final part of earthquake preparedness involves the formulation of public emergency plans, including escape routes, medical facilities, shelters, food and water supplies. It also includes personal planning, such as emergency supplies (food, water, shelter and warmth), escape routes from houses and offices, and communication strategies (with a focus on ones that don’t involve the cellular network, which may not be functioning after a large earthquake).
Media Attributions
- Figure 6.5.1 Steven Earle, CC BY 4.0
- Figure 6.5.2 Public domain image from Aagard, B. et al, (2014). Earthquake outlook for the San Francisco Bay region, 2014 to 2043. US Geological Survey, https://pubs.usgs.gov/fs/2016/3020/fs20163020.pdf
- Figure 6.5.3 Image from Innovation Center. (n.d.). Earthquake early warning. Ocean Networks Canada. https://www.oceannetworks.ca/. Used with permission.
- Figure 6.5.4 Pearl River Tower, public domain image by Brad Wilkins, via Wikimedia Commons, https://commons.wikimedia.org/wiki/File:2009_03_03_Pearl_River_Tower.jpg
- Figure 6.5.5 Photo by Steven Earle, CC BY 4.0
- Figure 6.5.6 Google Maps – street view
- Province of British Columbia. Seismic Mitigation Program (last updated May 11, 2021). https://www2.gov.bc.ca/gov/content/education-training/k-12/administration/capital/seismic-mitigation#progress ↵