1.2 Earth System Science
Steve Earle
Earth System Science is based on the premise that on our planet everything is connected to everything else via a complex web of energy and matter transfers. There is exchange of matter between rocky material of the geosphere and the hydrosphere, the biosphere and the atmosphere, and all of those systems are also connected with each other. This concept of interconnectivity dates back to 1926 with the publication (in Russian) of Vladimir Vernadsky’s “The Biosphere,”[1] but it only really started to develop and become well known in the west in the 1970s through the work and writing of British scientist James Lovelock.[2] The term Earth System Science was coined in 1983. In 2001 a number of organizations met to draft a declaration of Earth System Science, which is duplicated here in Box 1.3. The important parts of the 2001 Amsterdam Declaration are:
- The Earth behaves as an isolated system of parts connected by transfers of matter and energy and by feedbacks,
- That system is subject to change, and that change can be human caused,
- Such changes can cross critical thresholds leading the Earth System to start operating in a different way, and
- There is no precedent or analogue for the present high rate of change in the Earth System.
Box 1.3 Declaration on Earth System Science[3]
- The Earth System behaves as a single, self-regulating system comprised of physical, chemical, biological and human components, with complex interactions and feedbacks between the component parts.
- Global change is real and it is happening now. Human-driven changes to Earth’s land surface, oceans, coasts and atmosphere, and to biological diversity, are equal to some of the great forces of nature in their extent and impact.
- Global change cannot be understood in terms of a simple cause–effect paradigm. Human-driven changes cause multiple, complex effects that cascade through the Earth System.
- Earth-System dynamics are characterized by critical thresholds and abrupt changes. Human activities could inadvertently trigger such changes and potentially switch the Earth System to alternative modes of operation that may prove irreversible and less hospitable to humans and other forms of life.
- The nature of changes now occurring simultaneously in the Earth System, as well as their magnitudes and rates of change, are unprecedented. The Earth System is currently operating in a no-analogue state.
An overview of some of the interactions that take place in the Earth System is given on Figure 1.2.1. It is important to point out that this highly-simplified diagram shows only a small fraction of the important interactions, and that it excludes both the energy input from the sun and the role of humans. More on those later.
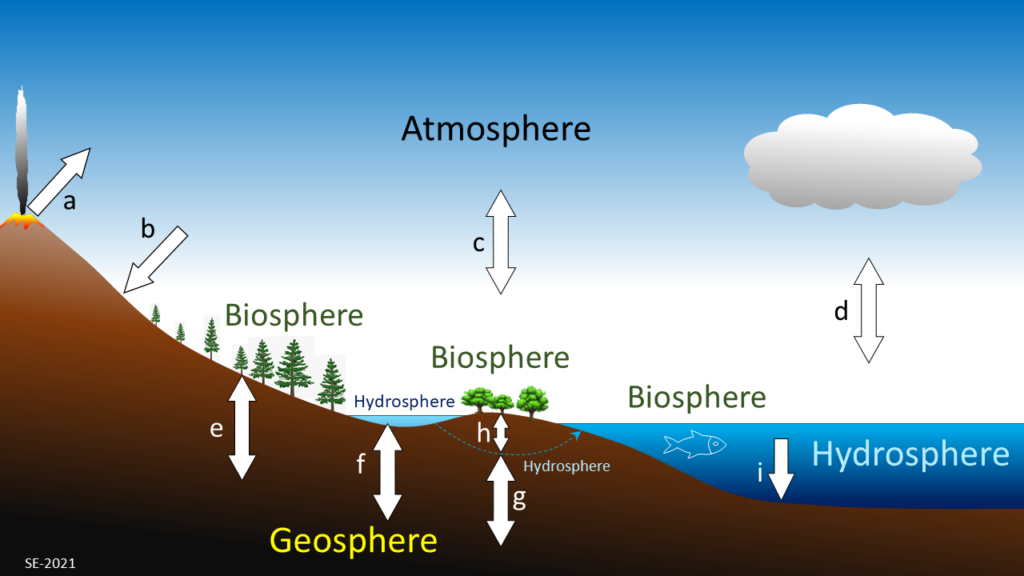
The single and double arrows of Figure 1.2.1 represent transfer and interchange of matter between the different parts of the system, but they don’t come close to revealing the complexity of the system because they should be shown as a series of intricate paths, twisting in and out, connecting each part of the system to almost every other part. For example, some of the water that originates by evaporation from the ocean rains down onto the ground, is modified by interactions with rock, and then is taken up by plants. Some of the chemicals from that water eventually come down as leaves and seeds and other plant parts and are then washed into the ocean where the chemicals are transferred into fish or marine plants. A fish swims upriver and is caught and partially eaten by a bear, the remains of the fish—which includes components from the ocean and the stream—fertilize the nearby trees. We could keep going on from there, but you probably get the point.
The labelled interactions of Figure 1.2.1 can be summarized as follows:
- During a volcanic eruption, gases (including H2O, CO2 and SO2) from the geosphere mix with the atmosphere, while volcanic rock fragments (ash) remains suspended in the atmosphere for a short time (weeks).
- Atmospheric gases (especially CO2, H2O and O2) react with rocks of the geosphere during weathering (an example is provided below).
- There is exchange of gases between the biosphere and the atmosphere as a result of photosynthesis in which CO2 in the atmosphere combines with H2O to produce glucose (C6H12O6) and O2, and respiration, in which glucose combines with O2 to produce CO2 and H2O and energy.
- There is exchange of water via evaporation and condensation, and of liquid water as rain, between the hydrosphere and the atmosphere.
- Components of the biosphere (e.g., plants) extract chemicals (e.g., phosphorous, potassium, magnesium, sulphur and calcium) from the geosphere, and in turn deposit material that gets incorporated into the geosphere.
- There are two-way chemical transfers between the rocks of the geosphere and the surface water of the hydrosphere (for example, some elements in rocks dissolve into surface water during weathering, and then may be deposited from surface water to form new rocks).
- There are two-way chemical transfers (including elements such as calcium, potassium, sodium, sulphur and carbon) between the rocks of the geosphere and the groundwater of the hydrosphere.
- There are two-way chemical transfers between groundwater and the biosphere.
- Components of the biosphere (especially carbon-bearing organic matter) and the hydrosphere accumulate as sediments (geosphere) on the floor of the ocean (and lakes).
These are just a few of the important interactions—there are many others—and it is critical to remember that all components of the Earth System are inter-related. No part of the natural system will operate on its own without input of matter and/or energy from some other part, and each component of the system has the potential to significantly influence the conditions in other components.
A good example of that interdependence is the relationship between the biosphere and the atmosphere. Photosynthetic plants (on both land and in the water) exchange gases with the atmosphere via photosynthesis and respiration as noted above. They cannot survive without atmospheric carbon dioxide and water, and in turn they influence the levels of atmospheric gases. As we’ll see in Chapter 3, photosynthesizers have been changing the composition of the atmosphere for over three billion years and are largely responsible for keeping our planet habitable.
Most of the interactions shown on Figure 1.2.1 involve movement of water. It is important to have some idea of the volumes of water that move through the different reservoirs, as shown on Figure 1.2.2. The majority of the evaporation (87% of it) is from the oceans, and the rest is from land and that includes water produced by plant transpiration. The majority of the precipitation falls onto the oceans (79%), while the rest falls onto land. The 8% difference between 87% and 79% is made up by river runoff from the land to the oceans and groundwater flow into the oceans. Most of the precipitation that falls on the land (88%) falls in “external runoff” areas where it can flow back to the oceans (in rivers and groundwater). The remaining 12% falls in dry areas of the continents—areas of “internal runoff”—and it is returned to the cycle only by evaporation.
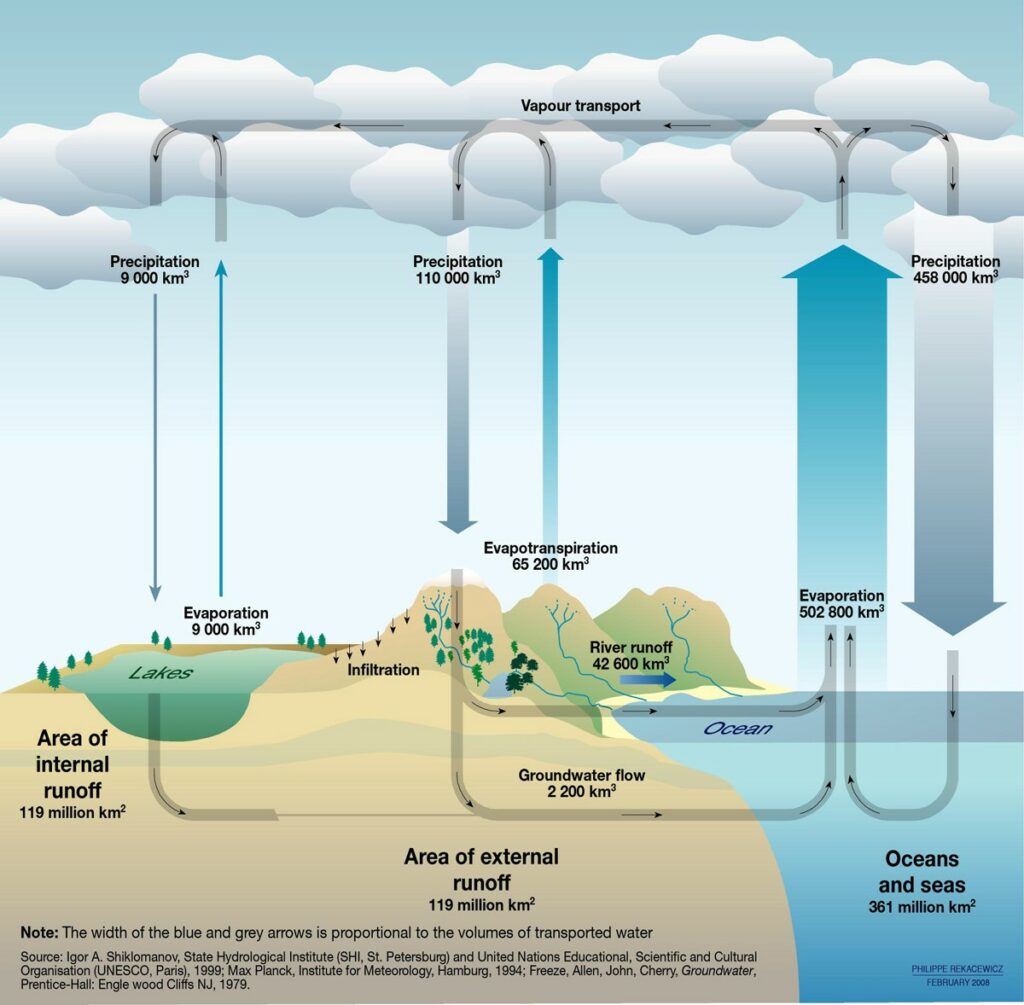
The water that is in the various reservoirs illustrated on Figure 1.2.2 stays in place for differing lengths of time, as summarized on Figure 1.2.3. For example, water in vegetation, the atmosphere, and rivers, typically stays there for days to weeks (although it can also be shorter or longer); for soil and lakes it may be years to decades; for glacial ice and the ocean it may be centuries to millennia; and for groundwater it might be as little as weeks, but it could also be decades to thousands of years, and even millions of years for some deep groundwater.
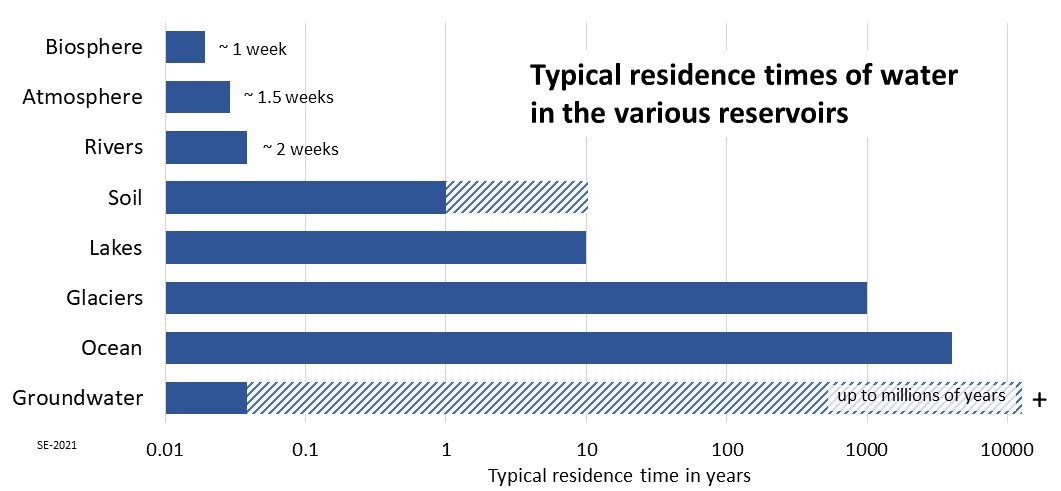
Exercise 1.1 Identifying Earth-System Interactions
Figure 1.2.4 shows Rubble Creek and its surroundings (near to Mt. Garibaldi, British Columbia). Identify as many of the interactions shown in Figure 1.2.1 (and listed below that figure) as you can.
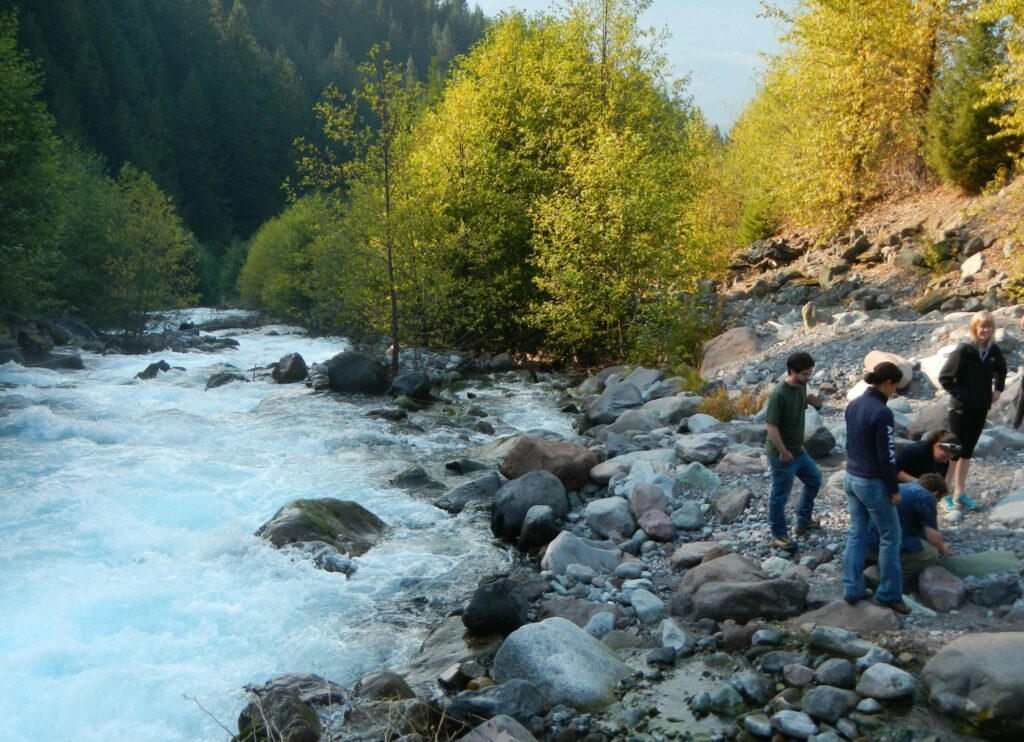
Exercise answers are provided in Appendix 2.
The sun, of course, is vitally important to the existence of life on Earth, but it also has a key role in many other Earth System processes. Some examples of the role of solar energy in Earth Systems are summarized on Figure 1.2.5. They include:
- Contributing to freeze-thaw and salt weathering by heating rock, and to weathering related to repeated expansion and contraction due to solar heating,
- Heating Earth surfaces (rock, soil, water, vegetation) which in turn emit infrared radiation, which adds heat to the atmosphere (via the greenhouse effect),
- Providing the radiative energy required for photosynthesis,
- Driving the hydrological cycle by evaporating water from lakes, oceans and vegetation, and
- Heating the near-surface ocean water, which generates wind (and sometimes massive storms as described in Chapter 14) and is a key driver for ocean currents.
Figure 1.2.5 Some Aspects of the Role of the Sun Within the Earth System
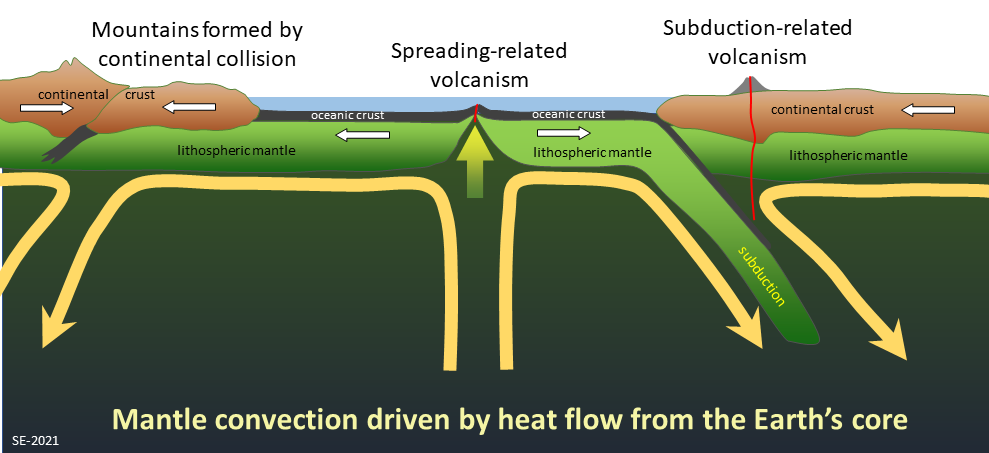
Earth’s systems are also influenced by what is happening deep within the Earth. Because of the significant size of the Earth, there is still a lot of heat left over from the collisions that took place during accumulation of fragments in its initial formation. Radioactive decay of elements like potassium, uranium, and thorium within the core also continue to contribute heat, and so the core is still very hot (up to about 7000⁰C—making it hotter than the surface of the sun) and partly liquid. This heat is slowly transferred from the inner core to the outer core and then from the core to the mantle, and that process leads to convection in the mantle (just like heat from your stove will cause convection in a pot of soup). In contrast, the Moon and Mars are much smaller than the Earth, and these bodies have lost enough heat to become too cold for continuing mantle convection. They don’t have plate tectonics, so mountain ranges are not being created, and they don’t have active volcanoes.[4]
As shown in Figure 1.2.6, convection in the mantle is the key driver of plate motions in the crust and uppermost mantle (although not the only one), and plate motions are associated with a number of features that have implications for Earth System processes. One of these is volcanism, which takes place in several different settings related to plate boundaries. Volcanism creates new crustal rock of course, but it also contributes gases to the atmosphere and water to the hydrosphere. Over geological time, volcanism has led to significant changes in the Earth’s atmosphere, and it may be the source of most of the water in the oceans. A large proportion of those gases and water come from the mantle, and some part of that is recycled back into the mantle during subduction, along with sediments that accumulated on the sea floor.
Plate boundary processes lead to the formation of mountains, both where continents collide (as India is colliding with Asia) and where there is subduction-related volcanism (like that in southern British Columbia, Washington, Oregon and northern California). Mountains are important in controlling many Earth Systems (e.g., weather patterns and biological processes). They also influence the composition of the atmosphere because steep slopes lead to accelerated weathering of rocks, and weathering of rocks consumes carbon dioxide through the process described below.
[latex]\ce{H2O + CO2 -> H2CO3}[/latex]
water + carbon dioxide[latex]\ce{->}[/latex]carbonic acid
Here we have water (e.g., as rain) plus carbon dioxide in the atmosphere, combining to form carbonic acid. The carbonic acid then combines with feldspar (the most abundant mineral in the crust) to form clay minerals along with dissolved ions through a process called hydrolysis. This process (which is illustrated in Box 3.1 of Chapter 3) can be written like this:
[latex]\ce{CaAl2Si2O8 + H2CO3 + 1/2O2 -> Al2Si2O5(OH)4 + Ca^2+ + CO3^2-}[/latex]
feldspar + carbonic acid + oxygen [latex]\ce{->}[/latex] kaolinite + calcium ions + carbonate ions
The kaolinite is relatively easily eroded by water and most ends up on the ocean floor, while the dissolved calcium and carbonate ions are also transported to the ocean where they will eventually be combined, with help from marine organisms, to form calcite.
Continents are moved by plate tectonic processes, and as described in Chapter 2, that can have implications for how much of the sun’s energy is converted to heat here on Earth. Ocean basins are also changed by plate tectonics, and that can affect the flow of ocean currents, a process which is critical to the redistribution of heat on the Earth.
Some of the Earth’s internal heat does reach the surface. The obvious manifestation of that is the heat from volcanic eruptions (Figure 1.2.7), but there is also a very slow flow of heat towards surface in non-volcanic areas. Contrary to what you might think, the warming of Earth’s surface is almost exclusively the work of the sun. The contribution of heat from inside is tiny, approximately 0.03% of the amount of heat that we get from the sun.
Finally, Earth Systems are significantly and increasingly influenced by human activities. A few of the relevant changes that we have made and activities that we undertake are illustrated on Figure 1.2.8. Those shown can be summarized as follows:
- Destruction of forests and other natural vegetation,
- Use of cleared land for crops and livestock,
- Use of chemicals (both fertilizers and pesticides) in agriculture,
- Use of cleared land for buildings, roads, parking lots and airports,
- Exploitation of petroleum and other fossil fuels and use of fossil fuels for transportation, electricity generation, farming, heating and manufacturing,
- Unsustainable and wasteful exploitation of marine food resources,
- Indiscriminate dumping of human and industrial wastes onto land and into water and the air,
- Disruption of natural surface water bodies (including drainage in some cases and flooding in others), and
- Changes to natural slopes to permit construction of buildings and roads.
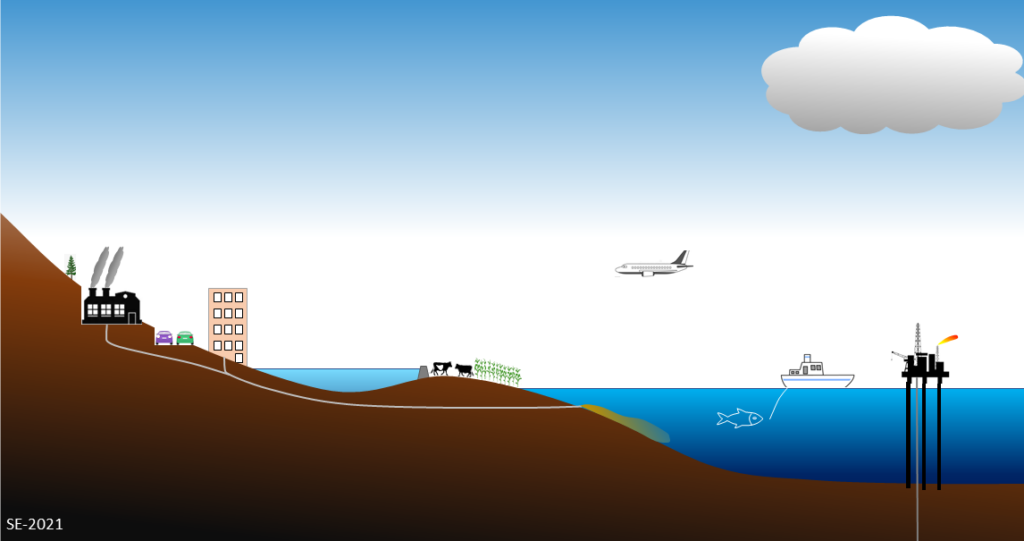
Exercise 1.2 Human Effects on Earth Systems
Figure 1.2.7 illustrates some of the changes that we have made to the Earth. Give an example of how each of the following might affect Earth Systems:
Action or Change | Impact on Earth Systems | Action or Change | Impact on Earth Systems |
Deforestation | Overfishing | ||
Farming | Waste disposal | ||
Constructing buildings and roads | Dams and dykes | ||
Using fossil fuels | Changes to natural slopes |
Exercise answers are provided in Appendix 2.
Another way to think about Earth System Science would be to consider any natural or man-made object on the surface of the Earth. Every natural object, say a bush, a bear, a boulder, or a body of water is obviously interacting (exchanging matter and energy) with its surroundings (water, rock, air, organisms). Every man-made object is doing the same thing, but in different ways. For example, an old car will interact with water and the air by rusting, and will release chemicals (e.g., dissolved iron and iron oxide minerals) into the environment. A concrete wall can be worn away by water or broken by a tree root, and can be chemically changed by oxygen, carbonic acid, and by plants. It can also release chemicals (e.g., calcium ions, clay minerals) into the environment. A piece of glass or plastic will eventually break down and start to fall apart, although that will take decades to centuries.
If you’re interested in how human constructions eventually succumb to Earth’s systems you might want to have a look at Alan Weisman’s book The World Without Us[5].
Field Trip 1.1
Time to get outside! Go for a walk anywhere near to where you live and find a man-made object that has been around for at least a few decades. It could be a concrete sidewalk, a road, a building, a gravestone, a statue, an abandoned vehicle, an old wharf, or a sea wall. (See Figure 1.2.9 for example)
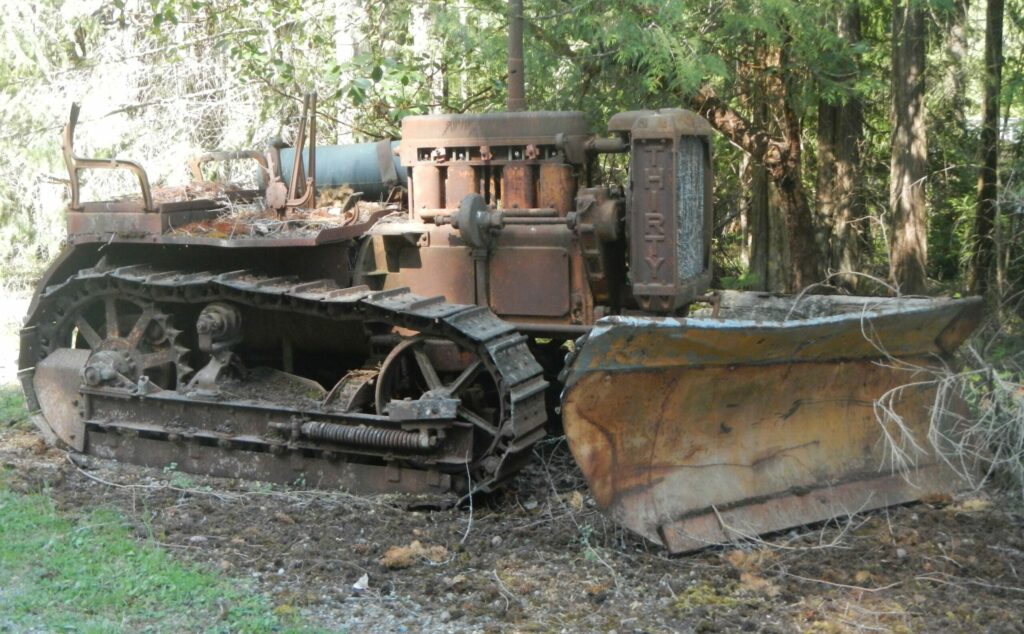
Try to estimate how long it has been there (there could be a date on it) and think about how it has been interacting with the oxygen and carbon dioxide of the atmosphere, the hydrosphere (rain, for example), the biosphere, and how it has been affected by the sun. As it starts to break down, how will the materials released affect the soil, the water, the air and the biosphere?
The soil around the tractor in the photo above is bare of vegetation. Can you think of a reason why?
A useful review paper on Earth System Science was published in 2020 by Steffen and co-authors[6]. They conclude with a statement on the importance of understanding Earth Systems now more than ever, because, in light of our changing climate, “humans are now the dominant force driving the trajectory of the Earth System.”
Media Attributions
- Figure 1.2.1 Steven Earle, CC BY 4.0
- Figure 1.2.2 Rekacewicz, P., and Digout, D. (2005). UNEP/GRID-Arendal. UN Environment Program (accessed May 2021). https://www.grida.no/resources/5794 Public domain,
- Figure 1.2.3 Steven Earle, CC BY 4.0
- Figure 1.2.4 Steven Earle, CC BY 4.0
- Figure 1.2.5 Steven Earle, CC BY 4.0
- Figure 1.2.6 Steven Earle, CC BY 4.0
- Figure 1.2.7 Steven Earle, CC BY 4.0
- Figure 1.2.8 Steven Earle, CC BY 4.0
- An annotated English translation of Vernadsky’s The Biosphere was published in 1998: Vernadsky, V.I. (1998). The Biosphere. Full translation of Vernadsky, V.I., 1926, The Biosphere. New York, NY: Copernicus Springer-Verlag. ↵
- See, for example, Lovelock, J. (1979). Gaia: A New Look at Life on Earth, Oxford University Press; and Lovelock, J. & Margulis, L. (1974). Atmospheric homeostasis by and for the biosphere: the Gaia hypothesis. Tellus, 26(1-2), 2–10. https://doi.org/10.1111/j.2153-3490.1974.tb01946.x ↵
- From the International Geosphere-Biosphere Program (accessed December 2020), http://www.igbp.net/about/history/2001amsterdamdeclarationonearthsystemscience.4.1b8ae20512db692f2a680001312.html ↵
- Lack of an internal heat engine may not be the only reason that the Moon and Mars don’t have plate tectonics as it is likely that the presence of liquid water at surface is an important aspect of that process, and neither of these bodies has that—at least not at present. There is more discussion of plate tectonics in Chapter 2. ↵
- Weisman, A. (2007). The World Without Us. Thomas Dunne Books/St. Martin's Press. ↵
- Steffen, W., Richardson, K., Rockström, J. et al. (2020). The emergence and evolution of Earth System Science. Nature Reviews Earth and Environment, 1, 54-63. https://doi.org/10.1038/s43017-019-0005-6 ↵