7.4 Volcanic Hazards
Steve Earle
There are two classes of volcanic hazards, direct and indirect. Direct hazards are those that can directly kill or injure people, or destroy property or wildlife habitat. Indirect hazards are volcanism-induced environmental changes that lead to distress, famine or habitat destruction. Indirect hazards of volcanism have accounted for many times more deaths during historical times than direct hazards. Some of the more important types of volcanic hazards are summarized in Table 7.4.1.
Type | Description | Risk |
Tephra emissions | Small particles of volcanic rock are emitted into the atmosphere | Respiration problems for some individuals. Short-term climate cooling and potential famine. Aircraft engines at risk. |
Gas emissions | The emission of gases during an eruption, or other event | Short-term climate cooling leading to crop failure and famine. Poisoning is widespread in some cases. |
Pyroclastic density current | A very hot (several 100°C) mixture of gases and volcanic tephra flows rapidly (up to 100s of km/h) down the side of a volcano | Extreme hazard. Virtually anything in the way will be destroyed. |
Pyroclastic fall | Vertical fall of tephra in the area surrounding an eruption | Areas close to the eruption (km to 10s of km) can be covered in thick tephra. Roofs may collapse; structures may burn. |
Lahar | A flow of mud and debris down a channel leading away from a volcano, triggered either by an eruption or a severe rain event | Anything within the channel will be severe risk. Lahar mud flows can move at 10s of km/h. |
Sector collapse/ debris avalanche | The failure of part of a volcano, either due to an eruption or for some reason, leading to an avalanche of debris | Anything in the past of the debris avalanche will be at severe risk. |
Lava flow | The flow of lava away from a volcanic vent | People and infrastructure are at risk, but lava flows tend to be slow (typically a few metres per hour on average) and relatively easy to avoid. |
Volcanic Gas and Tephra Emissions
Large volumes of tephra (rock fragments, mostly pumice, and volcanic ash) and gases are emitted during major explosive eruptions at composite volcanoes, and a large volume of gas is also released during some high volume effusive eruptions. One of the major implications of these emissions is cooling of the climate by up to 1˚ C for several months to a few years because the dust particles and tiny droplets and particles of sulphur compounds block the sun. The last time this happened was in 1991 and 1992 following the large eruption of Mt. Pinatubo in the Philippines. 1˚ C may not seem like a lot, but that was the global average amount of cooling, and cooling was more severe in some regions and at some times.
Over an 8-month period in 1783 and 1784 a large effusive eruption took place at the Laki volcano in Iceland. Although there was relatively little volcanic ash involved, a massive amount of sulphur dioxide was released into the atmosphere, and also a significant volume of hydrofluoric acid (HF). The sulphate aerosols that formed in the atmosphere led to dramatic cooling in the northern hemisphere. In Iceland poisoning from the HF resulted in the death of 80% of sheep, 50% of cattle, and the ensuing famine, along with HF poisoning, resulted in the over 10,000 human deaths, about 25% of the population. The Laki eruption also resulted in many deaths in Europe, although the total number isn’t known, it is estimated that there were approximately 20,000 deaths in the United Kingdom because of very cold weather,[1] and it seems likely that other parts of northern Europe would have been similarly affected.
Volcanic ash can also have serious implications for aircraft because it can destroy jet engines. In 2010 the eruption of Iceland’s Eyjafjallajökull volcano led to the closure of the European airspace for several days, and the cancellation of numerous trans-Atlantic flights.
Not all of the environmental effects of volcanism are related to eruptions of magma. The craters of dormant volcanoes are commonly filled with water (such as Crater Lake in Oregon). Within Lake Nyos in west central Cameroun, gases emanating from the underlying magma chamber continually percolate upward into the muddy lake sediment and bottom waters. One August night in 1986 a landslide, an earthquake or a minor eruption disturbed the lake sediment and the water and released approximately 100 million cubic metres of carbon dioxide from the lake bottom. The CO2 quickly bubbled up through the water and out into the air above the lake. The gas spilled over the lip of the crater and descended in a white cloud down into the valleys surrounding the crater. Over 1700 people and 3000 cattle were killed in their sleep.
There are other lakes in Africa with similar conditions, including nearby Lake Monoun, and the much larger Lake Kivu on the Congo-Rwanda border. An operation to reduce the risk of a future CO2 eruption from Lake Nyos is currently underway. The procedure involves lowering a strong polyethylene pipe to the lake bottom. Some water is pumped out at the top, and as the deep water rises through the pipe the carbon dioxide starts to bubble out. The gas and water then become buoyant and suck more water in at the bottom in a self-sustaining process (Figure 7.4.1).
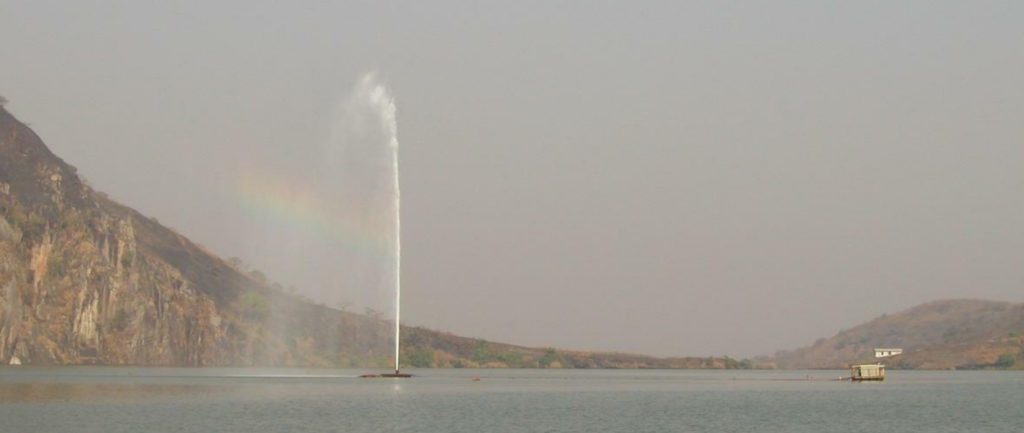
Pyroclastic Density Currents
In a typical explosive eruption at a composite volcano the tephra and gases are hot enough to be buoyant in the air and they are forced high up into the atmosphere. As the eruption proceeds, and the ejected materials start to cool, parts become heavier than air and they can then flow downward along the flanks of the volcano (Figure 7.4.2). As they descend, they cool more and so flow faster, reaching speeds up to several hundred km/h. A pyroclastic density current (PDC) consists of tephra ranging in size from microscopic shards of glass to boulders, plus gases (dominated by water vapour, but also including other gases). The temperature of this material can be as high as 1000˚ C. The most famous PDCs are the one that destroyed Pompeii in the year 79 AD, killing an estimated 18,000 and the one that destroyed the town of St. Pierre, Martinique in 1902, killing an estimated 30,000.
Pyroclastic density currents can flow over water, in some cases for tens of kilometres. The 1902 the St. Pierre PDC flowed out into the harbour and destroyed (burned) several wooden ships anchored there.
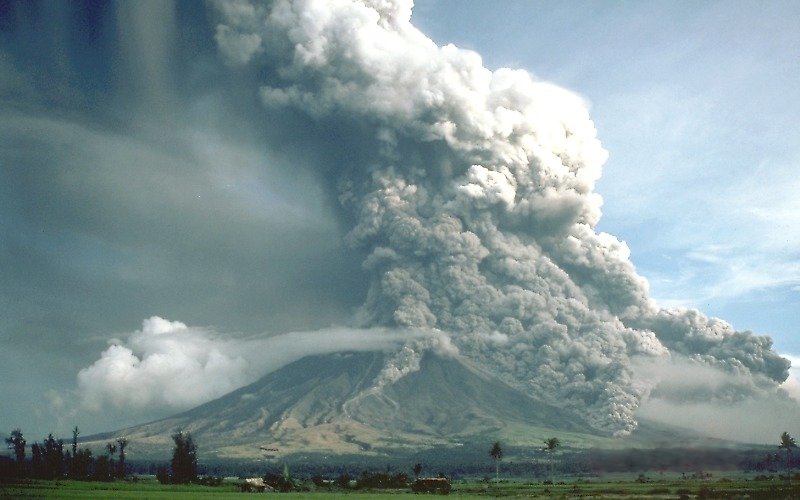
Pyroclastic Falls
Most of the tephra from an explosive eruption ascends high into the atmosphere, and some of it is distributed around the Earth by high altitude winds. The larger components (larger than 0.1 mm) tend to fall relatively close to the volcano, and the amount produced by large eruptions can cause serious damage and casualties. The large 1991 eruption of Mt. Pinatubo in the Philippines resulted in the accumulation of tens of centimetres of ash in fields and on rooftops in the surrounding populated region. Heavy typhoon rains that hit the island at the same time added to the weight of the tephra and led to the collapse of thousands of roofs and to at least 300 of the 700 deaths attributed to the eruption .
Lahars
A lahar is any mudflow or debris flow that is related to a volcano. Most are caused by melting snow and ice during an eruption, as was the case with the lahar that destroyed the Columbian city of Armero in 1985 (Figure 7.4.3). Lahars can also happen when there is no volcanic eruption, and one of the reasons is that, as we’ve seen, composite volcanoes tend to be weak and easily eroded.
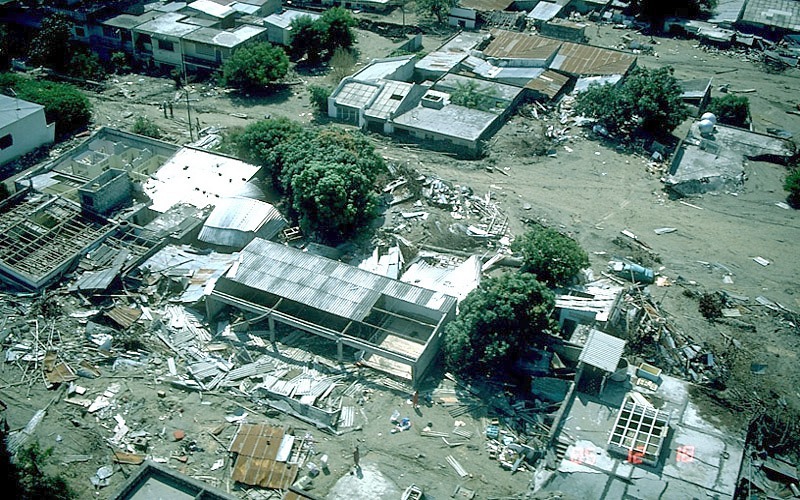
In October 1998 category 5 hurricane Mitch slammed into the coast of central America. Damage was extensive and 19,000 people died, not so much because of high winds but because of intense rainfall—some regions received almost 2 m of rain over a few days! Lahars (mudflows and debris flows) occurred in many areas, especially in Honduras and Nicaragua. An example is Casita Volcano in Nicaragua, where the heavy rains weakened rock and volcanic debris on the upper slopes, resulting in a debris flow that rapidly built in volume as it raced down the steep slope, and then ripped through the towns of El Porvenir and Rolando Rodriguez killing more than 2,000 people (Figure 7.4.4). El Porvenir and Rolando Rodriguez were new towns that had been built without planning approval in an area that was known to be at risk from lahars.
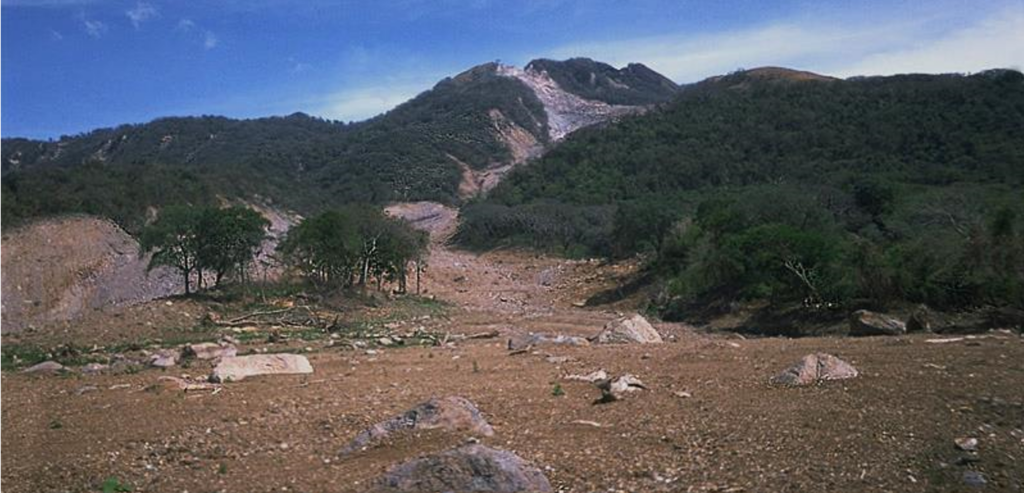
Central America was struck again by major tropical storms in November 2020. Hurricanes Eta (category 4) and Iota (category 5) also produced mudflows and lahars. The combined death toll in Central America was close to 200.
For several reasons, there are significant lahar risks from Washington State’s Mt. Rainier:
- It is the tallest and largest of the Cascade Range volcanoes,
- It has numerous large glaciers and accumulates a great deal of snow in winter,
- It has a significant volume of rock that has been weakened by alteration,
- It has been active within the past 125 years, and
- There are several large communities close to the mountain and within the channels of known past lahars (Figure 7.4.5).
According to a US Geological Survey (USGS) publication[2], Mt. Rainier is a lahar risk because there is a likelihood of melting snow and ice during an eruption, and also because there is a risk of slope failure at any time, but especially if there is a large earthquake in the region, or if there is movement of magma within the volcano itself. The USGS, Pierce County Department of Emergency Management, and Washington State Emergency Management Division have established an array of motion sensors in the drainage channels around Mt. Rainier. The system is designed to detect the vibrations associated with a lahar and then to alert at risk residents so that they have time to get to higher ground.
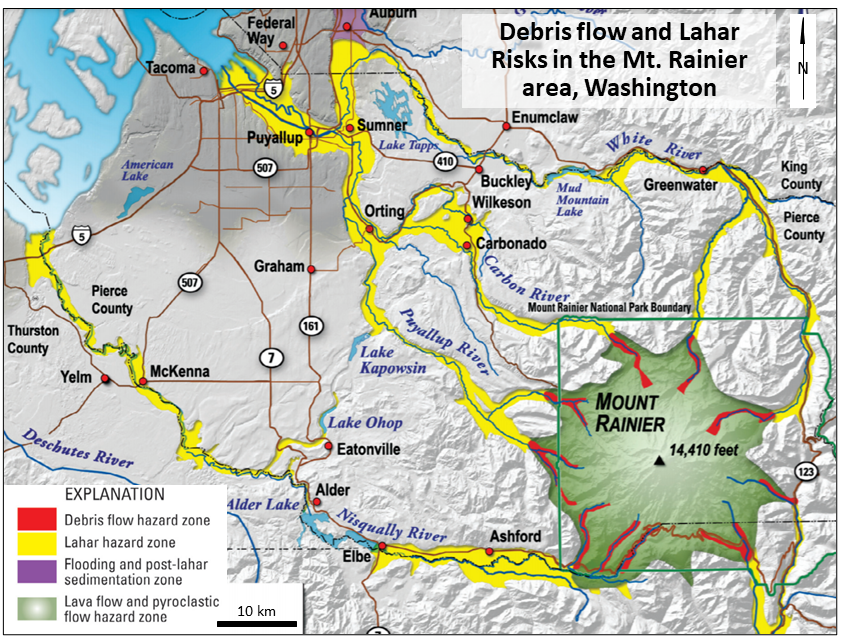
Sector Collapse and Debris Avalanches
In the context of volcanoes, sector collapse or flank collapse is the catastrophic failure of part of an existing volcano, and the formation of a large debris avalanche. The best known example of sector collapse is the failure of the northern side of Mt. St. Helens immediately prior to the large eruption on May 18, 1980. In the weeks leading up to the eruption a large bulge had formed on the side of the volcano, likely the result of transfer of magma from depth into a satellite magma chamber within the mountain itself. Early on the morning of May 18 a moderate earthquake struck nearby and this is thought to have destabilized the bulge, leading to the Earth’s largest slope failure in historical times. The failure of this part of the volcano exposed the underlying satellite magma chamber, causing it to explode sideways, and that exposed the conduit leading to the magma chamber below. The resulting eruption—with a 20 km high eruption column—lasted for 9 hours. Approximately 1 cubic km of tephra (volcanic rock fragments and ash) erupted, making this a relatively small eruption.
In August 2010 a large part of the side of BC’s Mt. Meager gave way, sending about 48 million cubic metres of rock down the valley, the largest slope failure in Canada in historical times (although the volume was only about 1/60th of the Mt. St. Helens 1980 sector collapse). This represents only a tiny fraction of the volume of Mt. Meager, but it can be considered a partial sector collapse. More than 25 slope failures have taken place at Mt. Meager in the past 8,000 years, some of them more than 10 times the volume of the 2010 failure.[3]
Lava Flows
As we’ve seen in Exercise 4.4, lava flows at volcanoes like Kilauea do not advance very quickly, and in most cases, people can get out of the way. Of course, it is more difficult to move infrastructure, and so buildings and roads are typically the main casualties of lava flows.
That was the case with the massive Kilauea eruption in 2018 where lava flowed through a mostly rural area (Figure 7.4.6), resulting in 24 injuries, no deaths, $800 million in damages, and the loss of over 800 homes as a well as a geothermal energy plant. But the situation at Mount Nyiragongo in the Republic of the Congo in 2002 was quite different. There a similar-sized lava stream flowed through Goma, a city of 200,000 people, destroying thousands of buildings, and leaving about 120,000 people homeless. A total of 245 people died, most from carbon-dioxide asphyxiation.
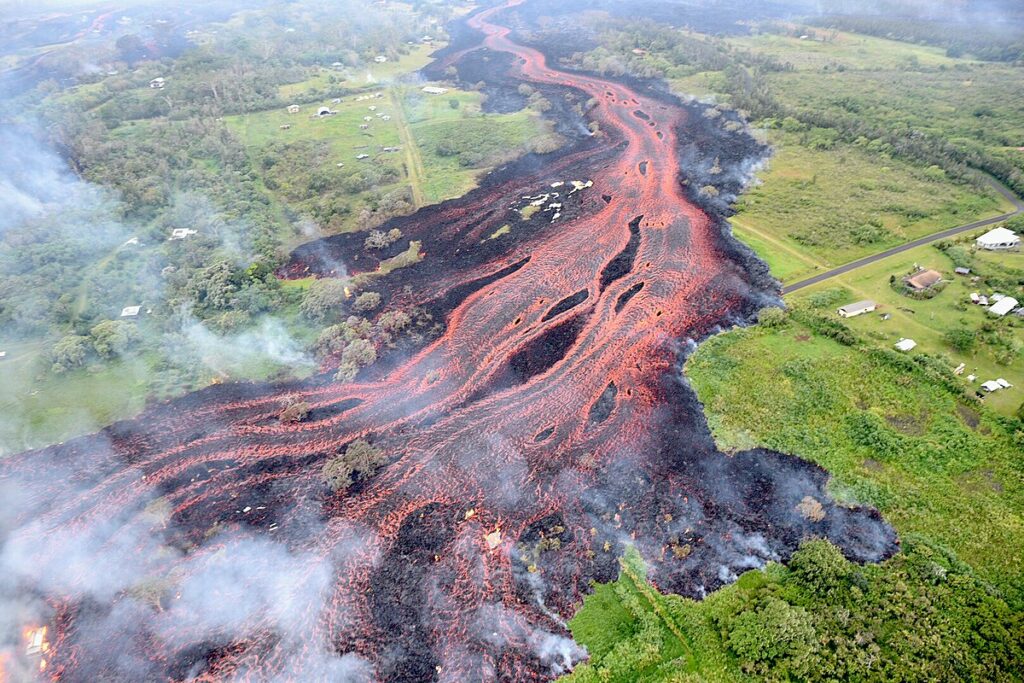
The eruption of the Tseax Cone in Northwestern BC, sometime between 1668 and 1714 resulted in a lava flow that now covers about 100 km2 (Figure 7.1.4). The flow destroyed two Nisga’a villages and killed about 2000 people, again, mostly as a result of carbon dioxide asphyxiation.
Exercise 7.5 Volcanic Hazards in Squamish
The town of Squamish, British Columbia, is situated approximately 10 km from Mt. Garibaldi, as shown on Figure 7.4.7. In the event of a major eruption of Garibaldi, which of the following hazards has the potential to be an issue for the residents of Squamish, or for those passing through on Highway 99?
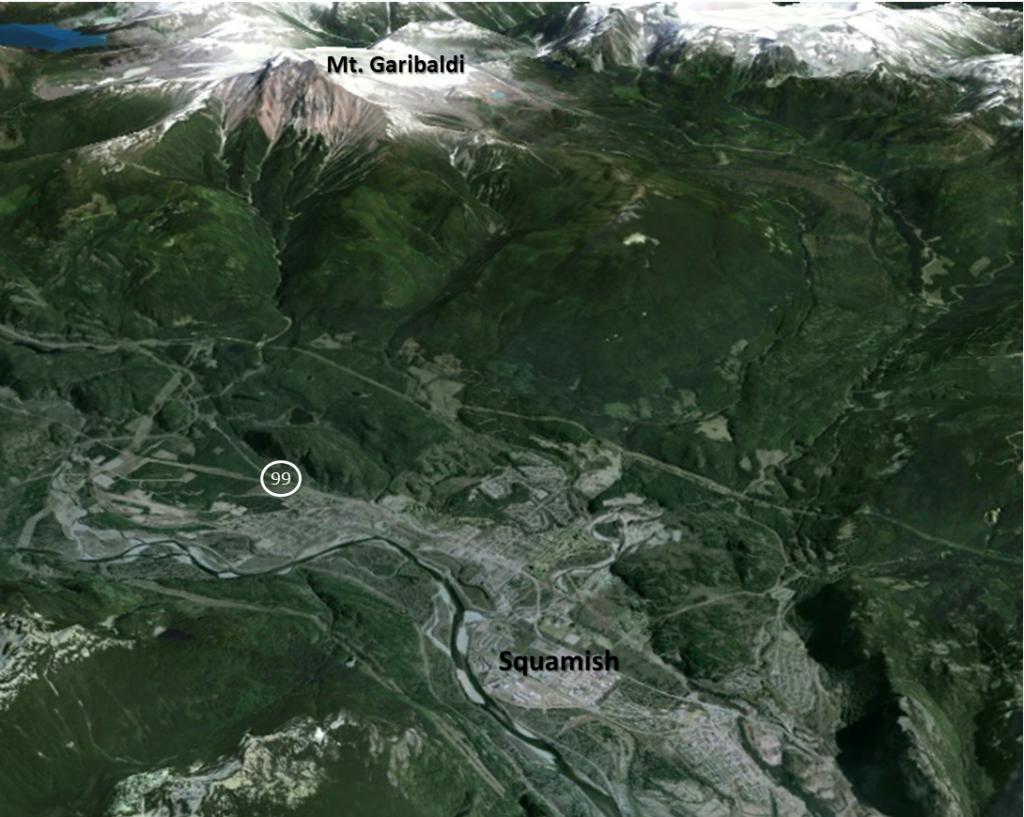
Hazard | Is it a risk in this area (yes/no) and brief explanation |
Tephra emission | |
Gas emission | |
Pyroclastic density current | |
Pyroclastic fall
Lahar Lava flow |
|
Sector collapse |
Exercise answers are provided Appendix 2.
Media Attributions
- Figure 7.4.1 Exploding Lakes in Cameroon, by Bill Evans, US Geological Survey photo, public domain. https://www.usgs.gov/media/images/exploding-lakes-cameroon
- Figure 7.4.2 Pyroclastic Flows at Mayon Volcano, by C. Newhall, US Geological Survey, public domain, via Wikipedia, https://en.wikipedia.org/wiki/File:Pyroclastic_flows_at_Mayon_Volcano.jpg)
- Figure 7.4.3 Armero Mudflow and Ruins by N. Banks, US Geological Survey, Public domain, via Wikimedia Commons, https://commons.wikimedia.org/wiki/File:Armero_Mudflow_and_ruins.jpg
- Figure 7.4.4 Lahar from Casita Volcano, US Geological Survey, Public domain, http://volcanoes.usgs.gov/hazards/lahar/casita.php
- Figure 7.4.5 Debris Flow and Lahar risks map, Public domain, from Driedger, C. & Scott, W. (2008). Mount Rainier: Living safely with a volcano in your backyard. US Geological Survey, Fact Sheet 2008-3062, p.3. https://doi.org/10.3133/fs20083062
- Figure 7.4.6 Kīlauea Volcano’s Lower East Rift Zone from US Geological Survey, 2018, public domain, via Wikimedia Commons, https://commons.wikimedia.org/wiki/File:USGS_K%C4%ABlauea_multimediaFile-2062.jpg
- Figure 7.4.7 Steven Earle, CC BY 4.0, based on a an image from Google Earth
- Witham, C. S., Oppenheimer, C. (2004). Mortality in England during the 1783–4 Laki Craters eruption. Bulletin of Volcanology 67, 15–26. https://doi.org/10.1007/s00445-004-0357-7 ↵
- Driedger, C. & Scott, W. (2008). Living safely with a volcano in your back yard. U.S. Geological Survey, Fact Sheet 2008-3062, p.4. https://pubs.usgs.gov/fs/2008/3062/fs2008-3062.pdf ↵
- Guthrie, R., et al., (2012). The 6 August 2010 Mount Meager rock slide-debris flow, Coast Mountains, British Columbia: characteristics, dynamics, and implications for hazard and risk assessment. Natural Hazards Earth Systems Science, 12, 1277–1294. https://volcanoes.usgs.gov/vsc/file_mngr/file-87/nhess-12-1277-2012-Meagerlandslide.pdf ↵